Researchers use laser to ‘photograph’ the forbidden passage of electrons
In quantum mechanics, particles can travel through areas that are forbidden according to classical physics, a phenomenon called tunnelling. Researchers at Aarhus University and ETH Zurich in Switzerland have now mapped precisely where the particle emerges after its passage.
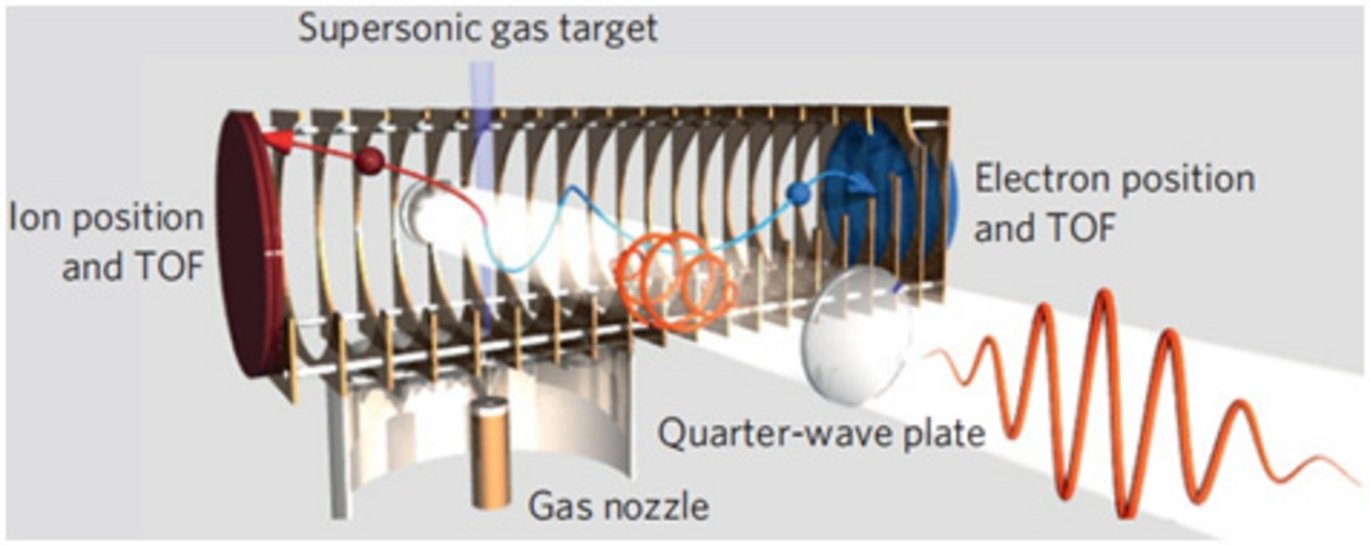
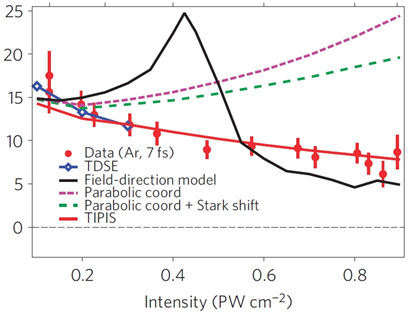
In quantum mechanics, weird and wonderful things happen that go against common sense. A particle can, for example, break through a potential barrier even though the barrier’s energy is greater than the particle’s binding energy. This phenomenon is known as tunnelling. Figuratively speaking, this corresponds to a ball being capable of rolling over a hill without having sufficient speed to get to the top.
Three years ago, the Swiss professor Ursula Keller and her team at ETH Zurich (the Swiss Federal Institute of Technology) discovered that tunnelling takes no time at all.
Collaboration between Aarhus University and the university in Zurich has now succeeded in getting even closer to this mysterious phenomenon. The two teams of physicists have mapped with great precision exactly where the particle – in this case an electron – emerges after tunnelling.
This collaboration began when the team in Switzerland asked physicists in Aarhus for help to explain the theory behind their experimental results. The Aarhus physicists took up the challenge and found an answer.
“The Aarhus theory takes a number of effects into account that have previously been omitted in mapping the exit point. We use the right ‘natural coordinates’, include the change in energy from the state before tunnelling, and take the multi-electron effect into account, i.e. the effect of the other bound electrons,” says Associate Professor Lars Bojer Madsen, Department of Physics and Astronomy, Aarhus University.
He developed the theory together with Postdoctoral Scholar Darko Dimitrovski and Postdoctoral Scholar Mahmoud Abu-samha, also from the Department of Physics and Astronomy.
The theory provides the most precise predictions to date of the particle’s exit point and has just been published in Nature Physics along with the experimental results.
A still shot of the electron’s passage using ultrashort laser pulses
The Swiss team ‘photographed’ the electron’s passage through the potential barrier using strong ultrashort laser pulses. The pulses are like a fast flash that gives the researchers a sequence of images of the electron’s passage.
In the new experiments, the use of circularly polarised light for the laser pulses instead of linearly polarised light is particularly revolutionary. It means that the electric and magnetic fields that constitute the electromagnetic waves we know as light circulate around the beam’s direction of propagation (see figure 1).
“The use of circularly polarised light is unique because it constantly drives the electron away from the atom, enabling us to isolate and determine its exit point. When using linearly polarised light, the electric field pulls the electron back when the direction of polarisation changes, and the returning electrons thereby pollute the measurements,” Dr Dimitrovski explains.
New theory contributes to improving attoscience
This new method is called the attoclock technique and it belongs to a branch of physics known as attoscience. Attoscience breaks down the movement of electrons along their natural timescale and scale of longitude. The name is related to the atomic timescale – attoseconds (10-18 seconds). It thus takes the electron in a hydrogen atom around 20 attoseconds to complete a circle around the nucleus.
The Swiss group from ETH Zurich is a world leader in experimental attoscience, and the university is among the absolute best in the world – with thirty-one Nobel Prize winners, including Albert Einstein.
“There are very few top experimental groups in the world, and Ursula Keller’s group is one of them. They could pick and choose between the groups of theorists, but they chose us because of the expertise we’ve developed in recent years in describing processes in circular fields,” the associate professor says.
He predicts that the new results will have a huge impact on the attoscience of the future.
“We provide a much more detailed understanding of the steps in the tunnelling process, which is vital for the development of light pulses and measuring techniques at the attosecond level,” he says.
For further information, please contact
- Lars Bojer Madsen, Department of Physics and Astronomy, Aarhus University, +45 8942 3674, bojer@phys.au.dk
- Darko Dimitrovski, Department of Physics and Astronomy, Aarhus University, +45 8942 3668, darkod@phys.au.dk
- Read the researchers’ summary of the results